How Deep Does Life Go?
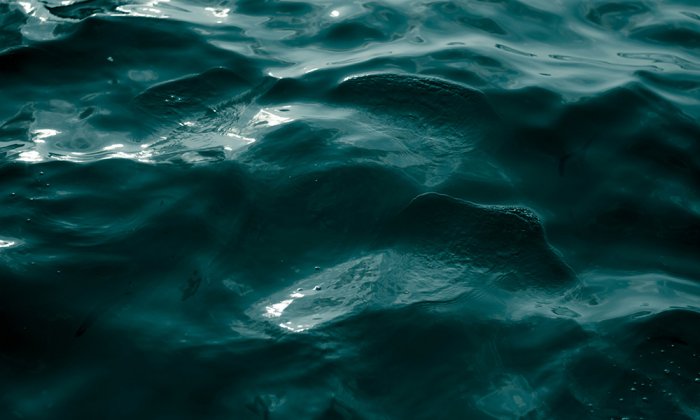
When HMS Challenger set sail in 1872, some scientists still believed in the azoic theory: that life cannot exist below 300 fathoms, or 550 meters. Others thought that creatures lived in the abyss, but that the cold and dark prevented them from evolving. With no more than their dredges, the Challenger scientists soon disproved both ideas.
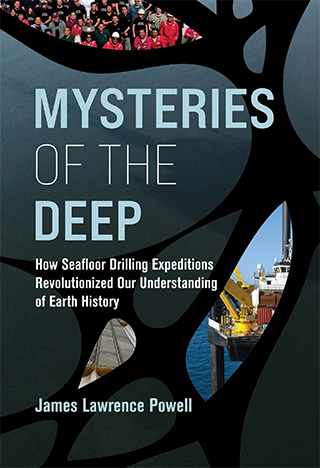
The exploration of life at and below the surface of the dark seafloor began with a 1936 article by Claude ZoBell and Quentin Anderson of the Scripps Institute of Oceanography, who found abundant bacteria in the surface layers of sediment cores 40 to 75 centimeters long taken off the coast of Southern California.
The deep sea and its creatures became a subject of great interest in the 1930s, prompted by the invention of the deep-sea submersible, a sort of mini submarine built to withstand the great pressures of the abyss. The most notable of these early vessels was the two-person “Bathysphere” used by famed scientist and author William Beebe (1877–1962), whose books with their photos of bizarre deep-sea creatures fascinated and inspired youngsters of an earlier time. Engineer Otis Barton designed the vessel and he and Beebe used it to make a number of deep dives off the coast of Bermuda. In 1934, the two reached a record depth of 923 meters.
The successor to Beebe’s Bathysphere was the Alvin, named for its inventor, the eponymous Al Vine, and launched in 1964 by the Woods Hole Oceanographic Institution. It was designed to carry two scientists and a pilot 4,500 meters down and allow them to stay at that depth for nine hours. Alvin made over 5,000 dives and fostered an estimated 2,000 research publications. But it had a rocky start, to say the least. Alvin’s first dive was in 1965 to 1,800 meters. In March 1966, Alvin was used in an unsuccessful attempt to recover a hydrogen bomb that had been lost in a midair accident and fallen to the seafloor at 910 meters depth off the coast of Spain. Then in October 1966, as Alvin was being lowered over the side of its support vessel, with crew members aboard and the hatch open, the two steel cables holding it broke. The crew was able to escape, but the vessel fell to the seafloor in 1,500 meters of water. The fortunate crew members had left their lunches behind, and when Alvin was hauled up, there the food was, intact and with no sign of attack by snacking microbes. This reinforced the view that the deep sea was inimical to significant bacterial life.
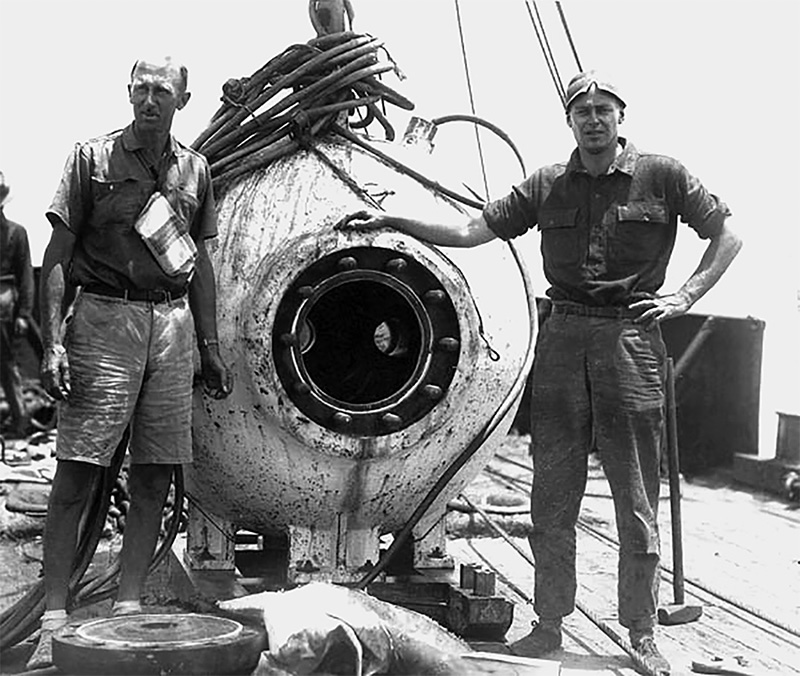
The Alvin’s most famous dive, however, was its 1986 exploration of the wreck of the ill-fated Titanic. After a complete overhaul, finished in 2014, Alvin was back on active duty at Woods Hole. In the summer of 2022, the submersible reached a record depth of 6,453 meters in the Puerto Rico Trench, meaning that Alvin could reach nearly any point on the seafloor.
Black Smokers
One of the key concepts of plate tectonics is that underneath the center of the oceanic ridges lie chambers of molten magma. These heat the adjacent seawater, which rises and flows out via hydrothermal vents. In 1977, scientists made 24 dives in the Alvin to study these vents along the Galápagos Rift, an offshoot of the East Pacific Rise. They found that two-thirds of the heat lost at the rift escapes via these outlets.
The scientists aboard Alvin found abundant life in a variety of forms on the Galápagos Rift at a depth far below that to which sunlight can penetrate.
Prior to these dives in the Alvin, scientists believed that photosynthesis was the ultimate and indispensable source of the energy needed to support life, meaning that living creatures could not exist in the blackness of the ocean depths. Yet the scientists aboard Alvin found abundant life in a variety of forms on the Galápagos Rift at a depth far below that to which sunlight can penetrate. Where did these creatures get the energy to sustain themselves if not from photosynthesis? Scientists had answered that phytoplankton, which consists of microscopic plants and lives near the surface, die and sift down as “marine snow,” a term coined by Beebe and a process recognized by the Challenger scientists. Rachel Carson described it in her book “The Sea Around Us”: “When I think of the floor of the deep sea … I see always the steady, unremitting, downward drift of materials from above, flake upon flake, layer upon layer — the most stupendous ‘snowfall’ the Earth has ever seen.” The dead phytoplankton fall to the dark, abyssal ocean floor and provide a food source for organisms living there. According to this theory, photosynthesis would still be the ultimate energy source for the creatures of the abyss.
But the hydrothermal vents on the Galápagos Rift held a concentration of organisms thousands of times greater than the seafloor around them. Some unrecognized process within the vents — not photosynthesis — was providing the energy on which the vent ecosystem depends. It turned out to be “chemosynthesis,” in which bacteria oxidize inorganic materials, primarily hydrogen sulfide, in chemical reactions that in turn provide the energy to sustain higher life forms. Black, irregular chimneys mark some vents where chemicals that had been dissolved in the hot water have precipitated as dark sulfides when the hot vent water meets the cold ocean. Scientists subsequently found these “black smokers” in many places in the Atlantic and Pacific Oceans, as well as stranded on land — for example, along the California coast where plate tectonics has lifted an old ocean floor well above sea level.
The creatures of the vent ecosystems ultimately depend on the sulfur-reducing bacteria — which we could almost say “breathe” sulfur — and include many strange denizens never before seen. None were stranger than the tube worms, which measure up to 3 meters long but are only 4 centimeters wide and live in clusters of thousands of individuals per square meter. They depend on the bacteria for energy and have no need for a digestive system. Their existence in such inhospitable conditions once again raised questions about what other life forms could exist at and below the seafloor.
Thomas Gold
If life can exist in the depths of the ocean, could a significant portion of all life on Earth be in those depths, rather than above them? That was the thesis of one of the most inventive and iconoclastic scientists of the second half of the 20th century. Thomas Gold was born in Austria in 1920 to Jewish parents who fled to England in 1938 after Hitler annexed Austria. Gold entered Trinity College at Cambridge, but when World War II broke out the British interned him as an enemy alien and deported him to a camp in Canada. After 15 months there, he was allowed to return to England, where he reentered Cambridge to study physics and worked on the all-important radar. Gold’s multifarious interests and accomplishments are enough to fill a book, or several. It is no surprise that he would be one of the first to explore the larger implications of the deep hydrothermal vents.
In a provocative 1992 article, “The Deep, Hot Biosphere,” and in a 1999 book of the same title, Gold extrapolated from the microbial life of the vents to propose that such life also existed in abundance beneath the seafloor. He went so far as to suggest that subsurface microbial life could be comparable in mass and volume to all life on the surface. Microbial life could be pervasive everywhere beneath Earth’s surface in the pore spaces between mineral grains — and not only on Earth but also on other bodies in the solar system: the Moon and Mars, for example. They have too little air and water to sustain life on their surfaces, but it could well exist below. Perhaps microbial subsurface life came first, protected from the surficial violence of the early solar system and using chemosynthesis, then evolved into photosynthetic life. Gold thought that microbial life might be widespread in the universe, a concept known as panspermia which goes back to the Greek philosopher Anaxagoras in the fifth century BCE. Many notable scientists have endorsed the idea, but with no way to test it, attention shifted to the possibility that the organic building blocks of life might have been present throughout the solar system at its beginning.
Drilling the Abyss
The detection of life beneath the seafloor was the goal of one of the earliest Deep Sea Drilling Projects (DSDP) voyages, Leg 15 in 1970, led by chief scientist Wallace Broecker of Columbia University. The crew found methane, a byproduct of microbial activity, in sediments 800 meters beneath the seafloor and tens of millions of years old. In October 1986, the crew of DSDP Leg 96 drilled the Mississippi Fan, a submarine pile of sediment in the northeastern Gulf of Mexico. They found subsurface microbial activity down to 167 meters beneath the seafloor. By the end of the century, the Ocean Drilling Program had sampled 14 sites for evidence of bacterial activity. A summary of these studies found that although the number of microbes typically decreases with depth beneath the seafloor, living cells are still present down to 700 meters. The authors came to the remarkable conclusion that the biomass in the top 500 meters of seafloor sediments equals 10 percent of that of the total surface biosphere. These early results suggested that living bacteria likely exist at greater depths than drilling had yet reached. This led to the first expedition designed specifically to study subsurface life.
In the spring of 2002, Ocean Drilling Program (ODP) Leg 201 drilled in two locations, one on the continental margin off Peru and the other in the equatorial Pacific. The subsurface ecosystems turned out to have a great diversity of microbes, including not only the sulfate-reducing bacteria found at the vents but a new type that got its energy from carbon reactions. The microbes were “alive” in that they engaged in metabolic activities such as repairing DNA and undergoing cell division. They included all three domains of life: archaea (one-celled organisms), bacteria, and eukaryotes (cells that have a nucleus). By this time, scientists estimated that subsurface bacterial life could amount to one-third that of Earth’s total living biomass. In 2003, ODP Leg 210 drilled the seafloor off Newfoundland and upped the ante once again. It found living bacterial cells 1,626 meters below the seafloor, in rocks 111 million years old, at temperatures of 113 degrees Celsius. This led the authors to estimate that bacteria in subsurface sediments may make up as much as two-thirds of total bacterial biomass.
The microbes were “alive” in that they engaged in metabolic activities such as repairing DNA and undergoing cell division.
In October 2010, expedition 329 of the Integrated Ocean Discovery Program (IODP), which followed the ODP, drilled in the South Pacific Gyre, some of the deepest water on Earth. It is the largest of the five giant oceanic systems of rotation that move enormous volumes of seawater. The South Pacific Gyre rotates counterclockwise, bounded by the equator to the north, Australia to the west, South America to the east, and the Antarctic Circumpolar Current to the south. Its center is the “oceanic pole of inaccessibility”: the location farthest from any continent. The South Pacific Gyre has one of the lowest sedimentation rates in the oceans and its bottom sediments have the lowest cell concentrations and the least metabolic activity of any. To discover the most extreme conditions under which life can exist on Earth, this is the place to go.
Aboard the JOIDES Resolution, still hard at work after all these years, in water nearly 6 kilometers deep, the scientists drilled 100 meters into the seafloor. They found microbes all the way to the bottom of the cores, albeit not as many as in the richer areas closer to the surface. The scientists estimated that the deepest microbes were at least 100 million years old, making it seem they could only be fossils. Surely nothing could “survive,” whatever that means exactly, for 100 million years. But when brought back to the lab and offered nutrients, the microbes began to grow and multiply.
This seemingly fantastic discovery raised the question of what the microbes beneath the gyre had been doing for 100 million years. Perhaps the cells had too little food to divide, but enough to repair damaged molecules. But that “seems insane,” said Steven D’Hondt, one of the leading authorities on microbial life in the seafloor, who wondered whether there is not another undiscovered source of energy — possibly radioactivity — that could support slow cell division.
On Expedition 337 of the IODP, the Japanese drilling ship Chikyū (Earth), designed for deep-sea drilling, cored to a depth of 2,466 meters beneath the seafloor off Japan’s Shimokita Peninsula. It found microorganisms in coal and shale that resemble those in the soil of modern tropical forests. These microbial communities are thought to be relics of those that inhabited soils about 20 million years ago, rather than more modern microbes that might have migrated into the coal layers from elsewhere. To explore the upper temperature limit at which microbes can survive, on Leg 370 of the IODP, Chikyū drilled in the Nankai Trough subduction zone off Cape Muroto in south-central Japan. The drill reached 4,776 meters and the deepest core was collected at 1,177 meters, where the temperature measured 120 degrees Celsius. Microbial life was detected all the way to the bottom of the sediment column. The cells at that depth appeared to spend most of their energy repairing the damage caused by the high temperature. Several authorities had written that the temperature limit to life in the subsurface was 80 degrees Celsius, but Gold had predicted that the upper temperature limit on bacterial life would be in the range of 120 to 150 degrees Celsius — and he turned out to be right.
Martians
These findings from scientific ocean exploration suggest that microbial life may be pervasive everywhere beneath Earth’s surface under conditions long thought to be inhospitable, if not fatal. This raises the possibility that, as Gold postulated, bacterial life may have existed and may still exist on other bodies in the solar system, including Mars. This despite the Red Planet’s hellish surface conditions, continually blasted by lethal radiation from the Sun and the cosmos. The surface temperature of Mars averages negative 60 degrees Celsius and is so dry that a cup of water would vaporize instantly. A group of scientists experimented with a terrestrial bacterium called Deinococcus radiodurans, said to be the toughest on Earth according to Guinness World Records, to test whether it could survive on Mars. This creature thrives in nuclear reactors. They found that if buried 9 meters underground, D. radiodurans could withstand Martian levels of radiation for 280 million years.
Later this decade, the European Space Agency plans to send a spacecraft to Mars that will drill more than 2 meters below the surface and analyze the organic molecules found there. How will we humans react if, when life is discovered on another planet, it looks nothing like us, nor even little green men, but is microbial? If evidence of microbial subsurface life is found on Mars, it may have been the first lifeform in the solar system, where, protected from the surficial violence and using chemosynthesis, it could indeed have evolved into photosynthetic life and eventually, us. If Earth and Mars harbor subsurface bacterial life, why not other planets as well?
James Powell is a retired geologist and university administrator. He is the author of several books, including “The Inquisition of Climate Science” (Columbia University Press), “The 2084 Report: An Oral History of the Great Warming” (Simon & Schuster), and “Mysteries of the Deep,” from which this article is excerpted.
Correction: An earlier version of this article stated that Alvin’s most famous dive was its 1968 exploration of the wreck of the Titanic. The Alvin explored the wreckage in 1986.